A fish ladder to help fish swim upstream
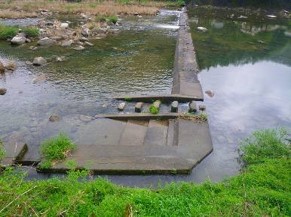
Photo 1 Stepped fish ladder
installed in a weir
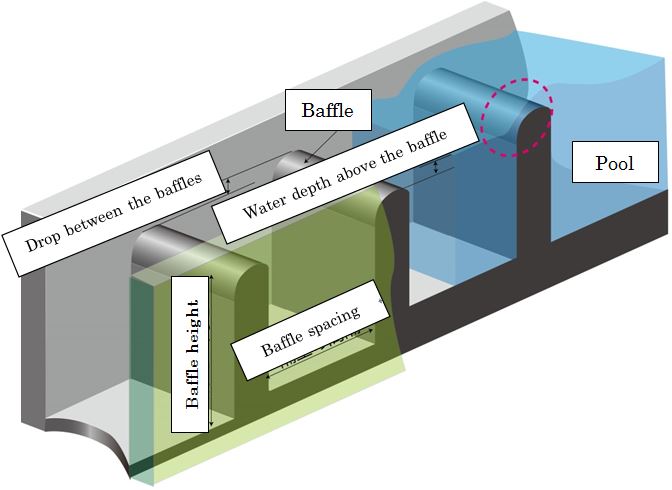
Figure 1 Construction of a stepped
fish ladder
(click to enlarge)
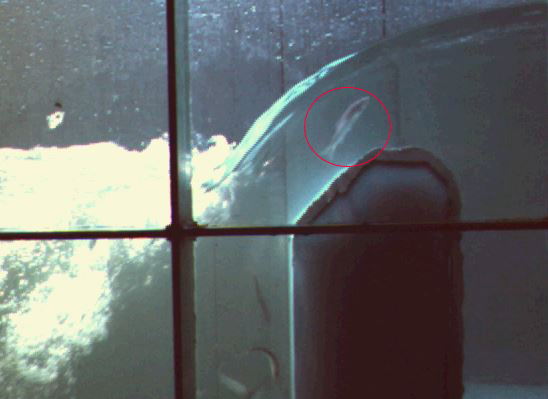
Photo 2 Sweetfish passing over
a baffle (Baffle A)
(Click on image to start video)
Many species of fish, varying both in size and in the way they swim, live in rivers. Over the course of their life, species will typically migrate between downstream and upstream river areas, or between rice paddy areas and ponds in the vicinity of rivers. However, in many rivers there are structures designed to support human activities, which may restrict the movement of fish. Fish ladders are sometimes installed in such structures, to allow fish to move between downstream and upstream areas (Photo 1). A stepped fish ladder, the most common type of fish ladder in Japan, with a history dating back to the Meiji Era, consists of a series of baffles and mini-pools to lessen the drop between larger pools (Figure 1). At a glance, it seems like a simple construction, but the facility with which fish can swim up the ladder depends on a complex combination of the height and spacing of the baffles, the drop between the baffles, and the water depth above individual baffles. For example, in such a ladder, with an increase in the drop between two baffles, the water velocity increases in the area where the water flows from the top of the upper baffle into the lower pool (indicated by the red dashed circle in Figure 1). Therefore, improvements are sometimes made, to reduce the drop, such that small fish with less power can swim upstream. However, a study by the PWRI showed that, with the same drop, even small improvements can make it easier for fish to swim upstream.
Photo 2 is a video of a sweetfish passing over a baffle with a baffle-to-baffle drop of 15 cm, which was taken with a high-speed video camera (water depth above the baffle: 20 cm). The event is only 0.5 seconds in duration, but, as shown in the video, it is not easy for fish to swim over the baffle. When the trajectories of sweetfish passing over the baffle under the same conditions are superimposed, it can be seen that most of the sweetfish are pushed back downstream for a short period of time, in the same location, whether or not they ultimately succeed in passing over the baffle (left and middle in Figure 2). Analysis of fish swimming speed and other data showed that the flow direction relative to the direction of travel suddenly changed in a location where the inclination of the baffle top changed sharply (indicated by the arrow ↑ in Figure 2), and that this may have thrown the fish off balance. In Baffle B (right side of Figure 2), where the baffle inclination changed smoothly, only a small number of fish lost balance, and the average time required for fish to pass over the baffle was less than half that for Baffle A. In addition, it has been found that such sudden changes in water flow can affect the migration of bottom fish, such as sculpin, as well as sweetfish.
Here, we have focused on the ease of passing over the baffle. Ease of approaching the baffle requires consideration from a different perspective. We will develop various improvements for other types of fish ladders, such that, in each case, fish can easily swim up the ladder.
(Contact:River Restoration Research Team)
Elucidating the Mechanism of Long-term Ground Subsidence in Peaty Farmland
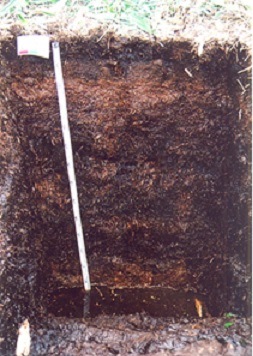
Figure 1 Cross-section of peat soil
(Plant fibers are observable.)
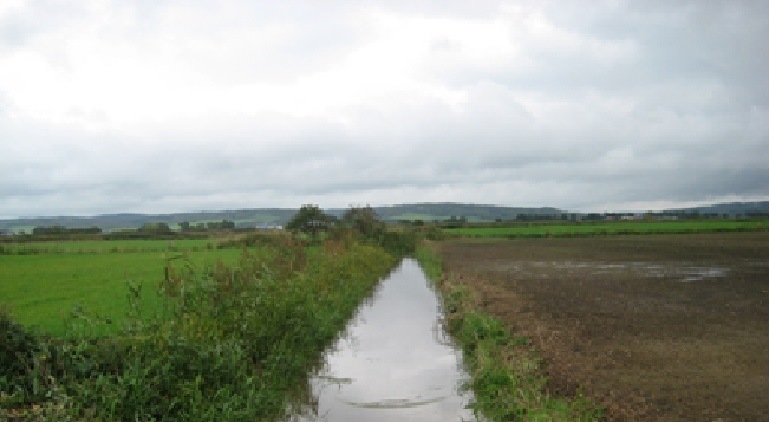
Figure 2 Ground subsidence around
the drainage ditch has made
the ditch shallower, and water
has pooled on the farmland.
(click to enlarge)
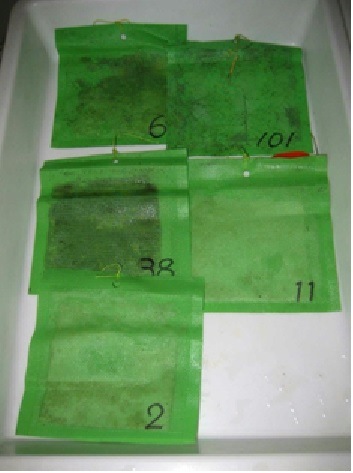
Figure 3 Bags filled with organic
matter and used for
estimating ground subsidence
The CERI Rural Resources Conservation Research Team has been conducting research on the mechanism of long-term soil subsidence in peaty farmland as well as on techniques for controlling such subsidence.
Peat consists of incompletely decomposed plant remains that have accumulated in a water-saturated environment, such as a marsh. The plant fibers in peat soil are visible to the naked eye. Because peat is spongy and highly compressible, it is not easy to stand steadily on peaty ground. One who steps onto such ground might even sink to the waist in peat. Peaty land tends to be flat and distributed at the middle and lower reaches of the river basin, where irrigation water is readily available. Peaty land is often used for farming.
Before peaty land can be farmed, it needs to be drained. The ground gradually starts to subside after it is drained. As ground subsidence progresses, a relative rise in the groundwater level causes agricultural productivity to decrease because crops are damaged by excessive moisture. To prevent damage to crops, it is necessary to apply soil dressing to the farmland or to ditch the farmland again in order to lower the groundwater level. The farmland owner has to bear an additional financial burden.
In farmland that was developed on peaty land in 1961, ground subsidence began immediately after the completion of the farmland development and it has continued to date. Measurements of the ground surface elevation indicate a cumulative ground subsidence of more than 1.5m on average. Drilling log records of the current farmland show the void ratio of the peat to a depth of 1 m from the surface to be smaller than the void ratio of the underlying peat. This difference in void ratio was not observed in drilling log records of the farmland in 1961. It is obvious that the peat distributed to a depth of 1 m was consolidated.
Based on the observation results described above, the mechanism of ground subsidence is assumed to be as follows. 1) The groundwater level is lowered by drainage of the peaty soil. 2) Ground subsidence is caused by the natural dehydration of any peat that is higher than the groundwater. 3) Dehydration (i.e., unsaturation) causes peat to compact. 4) Ground subsidence is caused by the consolidation of any saturated peat that is lower than the groundwater.
Additionally, it is assumed that ground subsidence is also caused by the decomposition of plant fibers, which is accelerated when fibers that have been submerged are exposed to air after the groundwater level has decreased. To test this assumption, the amount of ground subsidence resulting from the decomposition of plant fibers was estimated. To estimate the magnitude of ground subsidence caused by the accelerated decomposition of plant fibers, bags filled with organic matter were buried in peaty ground. The rate of decomposition was estimated by measuring the lost weight of the bags. The annual ground subsidence estimated on the basis of the measurement was 1.8 mm; thus, ground subsidence resulting from the decomposition of plant fibers is likely to account for an insignificant part of peaty ground subsidence.
With a view to maintaining productive farmland as well as to facilitating sustainable food production and farm management, CERI has been developing techniques for controlling the subsidence of peaty farmland. On the basis of long-term observation data and experiment results, CERI has been analyzing how subsurface drainage affects peaty ground subsidence and to what extent peaty ground subsidence can be controlled by raising the groundwater level while farmland is not being used for production.
(Contact:Rural Resources Conservation Research Team,Civil Engineering Research Institute for Cold Region)
Field Verifications of a Method for Evaluating Coastal Structures in Terms of Their Function to Create Seaweed Bed Formation:A Proposed Method for Diagnosing the Function of Environmental-harmony Structures to Create Seaweed Bed Formation by Incorporating the Concept of Stock Management
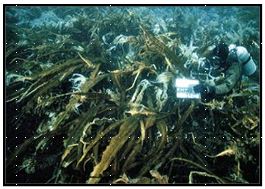
Seaweed in the initial phase
of construction
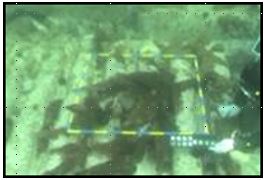
Seaweed a few years after
construction
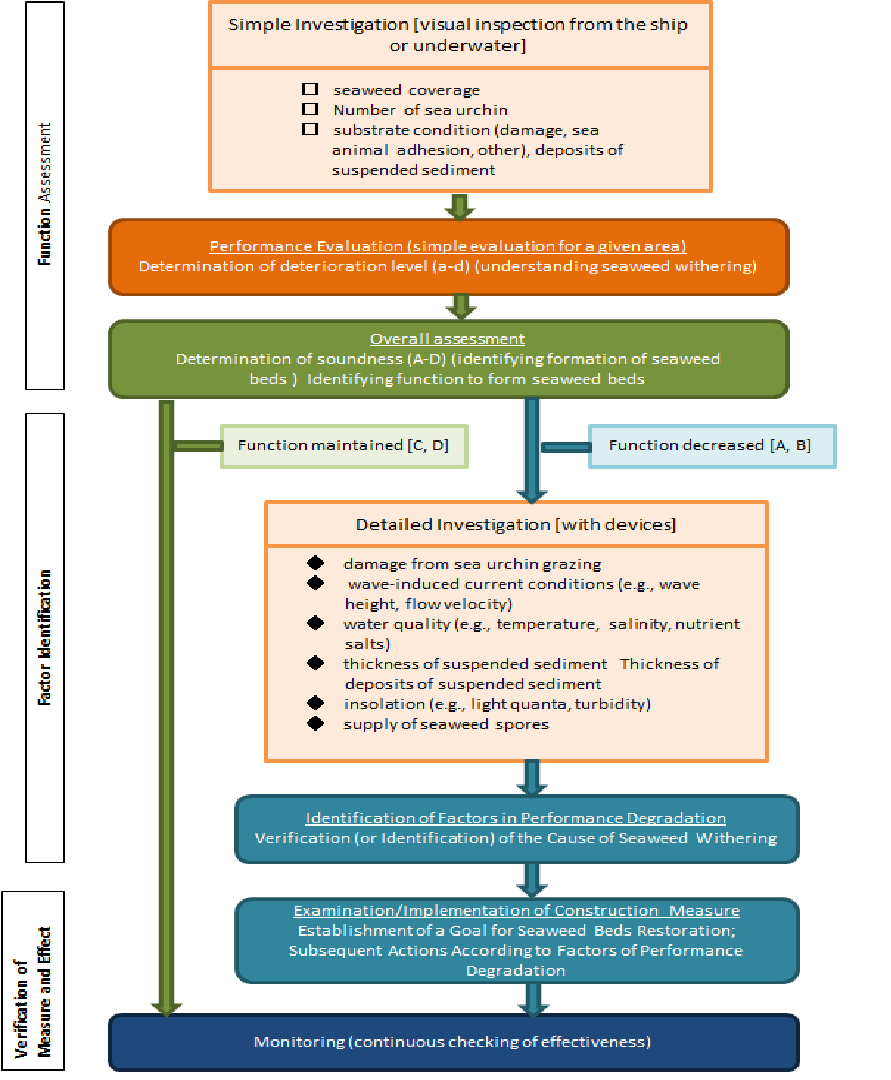
Proposed scheme for diagnosis of
(click to enlarge)
The waters around harbors, fishing ports and coastal structures are important habitats for marine life, as well as being spawning and rearing grounds for such life. With some ingenuity, artificial structures can be made to achieve harmony with the environment. Specifically, they can be made to purify water, create the formation of seaweed beds and protect fish spawning grounds and habitats of juvenile fish. In Hokkaido, seaweed beds have been created on breakwaters and revetments, with the aim of making coastal structures more environmental-harmony.
However, it has been revealed that some artificial structures at some sites have not achieved harmony with the environment. At such sites, the function of seaweed beds to form was found to be impaired. This is partly because of habitat changes caused by global warming and by decreases in nutrient salts, and because of seaweed withering due to increases in grazing pressure by sea urchins and other herbivores. Unfortunately, no systematic investigation has been conducted to clarify the association between the above phenomena and impairment of the function of structures to create seaweed bed formation.
Essentially, an approach incorporating the concept of stock management should be taken to maintain function to create seaweed bed formation. The approach includes two main steps: assessment (or diagnosis), and restoration. In the assessment step, a given structure is assessed in terms of how well it demonstrates its initially expected function to create seaweed bed formation. In the restoration step, the factors that inhibit that function are identified and remedial measures are taken. In fact, many methods for diagnosing function that focus on concrete cracking and steel corrosion of structures have been proposed from physical or scientific perspectives. These methods, however, do not lead to the maintenance of the structure's function to create seaweed bed formation, because they do not include a systematic method for assessing the soundness of the marine ecology.
The Civil Engineering Research Institute for Cold Region has examined methods for maintaining environmentally harmony coastal structures such as to meet the changing environment, and for evaluating the function of such structures. We have also been testing those methods onsite. Our studies have found that, in evaluating a structure's function to create seaweed bed formation, an understanding of the patterns of seaweed withering is essential. They have also found that rough evaluation may be possible by studying seaweed growth and damage (or feeding pressure) caused by feeding animals. In the next step, we identified indicators of the influence of structures on seaweed withering, and we propose a method for diagnosing the function of these structures to create seaweed bed formation by using deterioration indices including "seaweed coverage" and "sea urchin distribution density." We also tested the method on existing structures and verified its appropriateness. We will conduct further tests, upgrade the evaluation items and indices, improve the evaluation accuracy and increase the versatility of the method.
(Contact:Fisheries Engineering Research Team,Civil Engineering Research Institute for Cold Region)