Study on Long-Term Quality Management Technologies for Construction Generated Soil
The soil generated from ground excavation and other work associated with construction is called "construction generated soil." Most construction generated soil is safe and free of contamination. However, given that excavating natural mountains to obtain new soil needed for construction is environmentally burdensome, and that the disposal of construction generated soil puts pressure on available waste disposals spaces, the effective use of construction generated soil at other construction is required.
In the past, road embankments and river levees were constructed by purchasing good-quality sand or using only the good-quality portions of construction generated soil. However, recently, the application of stabilization1 and other technical innovations has led to the use of low-quality construction generated soil2 that was previously disposed of.
PWRI is conducting joint research with a total of twelve people in industry and academia on quality management technologies for ensuring that road embankments constructed with low-quality construction generated soil demonstrate long-term stability and durability. Specifically, we are conducting strength checks through a boring survey of a road embankment for which approximately ten years have passed following construction (Photo 1) and long-term exposure tests on stabilized soils that simulate severe environments indoors (Photo 2). The purpose is to clarify how water in such forms as torrential rains, long-term rainfall, and groundwater affects the strength of the stabilized soils. Additionally, we are conducting studies toward improving construction and quality management technologies with a view to long-term maintenance and management by simulating compaction of actual-size embankments in a concrete pit measuring some 50 meters in length (Photo 3).
We aim to promote the safe recycling of low-quality construction generated soil through this study.
Note 1: "Stabilization" technology refers to the mixing of stabilizing material such as lime or cement into soil to improve its quality by consuming moisture and creating solidified material (hydrate) through the chemical reaction of moisture in the soil, the stabilizing material and soluble component of the soil.
Note 2: "Low-quality construction generated soil" refers to soil with insufficient soil strength for use in embankments and other structure as well as soil with a high moisture content that many construction machines have difficulty handling.
(Contact: Construction Technology Research Team)
Development of Methods for Monitoring Zooplankton and Phytoplankton using a Next-Generation Sequencer in Dam Reservoirs
Introduction
The monitoring of zooplankton and phytoplankton in dam reservoirs is conventionally done based on the "Manual for National Census on River Environments (Dam Reservoirs) V. Zooplankton/Phytoplankton Surveys," and their identification is made through a microscopic examination using an optical microscope. However, identification requires advanced skills which are available only to limited number of technicians. Moreover, because identification is made based on form, determining zooplankton and phytoplankton that have very similar forms can be difficult.
On the other hand, recent rapid developments in genome analysis technology are making it easier to acquire large-scale DNA base sequence data using a next-generation sequencer (hereinafter "NGS"). It is expected that such data will be applicable to the identification of zooplankton and phytoplankton.
In this study, we are establishing a monitoring analysis method based on DNA base sequences that uses an NGS. Our aim is to develop an effective zooplankton/phytoplankton monitoring method for water quality management in dam reservoirs.
2. Monitoring trial using water samples from dam reservoirs
We collected surface water samples from four different dam reservoirs (A to D) and detected zooplankton and phytoplankton in them on a monthly basis. An NGS (MiSeq) made by Illumina was used to determine base sequences. For the detection of zooplankton and phytoplankton, we used primers targeting the 18S rRNA gene region (V4-V5 region).
One hundred and seven zooplankton and phytoplankton species were identified as closely related species. In the National Census on River Environments, between approximately 30 and 70 species of plankton were identified using an optical microscope in the same dam reservoirs. The results we obtained using the NGS covered almost all of these species .
The results of zooplankton and phytoplankton detection in each dam reservoir (phylum level) are shown in Fig. 1. Dominant species varied from reservoir to reservoir, and shifts in dominant species were observed throughout the year. At Dam A, the percentage of ciliates increased from January to March, while at Dam B the detected percentage of Ochrophyta was comparatively large. At Dam C, dinoflagellates, which may cause freshwater red tide, were dominant from September to November, while at Dam D, the detected percentage of green algae was large from May to July. Additionally, while not shown in the figure, detected plankton species were also found to vary greatly at deeper and deeper depths.
3. Conclusion
We intend to conduct more detailed studies in the future towards a practical application of a monitoring method using an NGS, thus contributing to a better water quality management for dam reservoirs.
(Contact: Water Quality Research Team)
Study on Assessing the Durability of RC Deck Slabs for Highway Bridges
- Based on an Analysis of Deteriorated Highway Bridge Members and Their Deterioration Conditions in a Cold Region -
Of the various highway bridge construction members, reinforced concrete (RC) deck slabs are the most susceptible to deterioration and damage. Particularly in cold regions such as Hokkaido, RC deck slabs degrade easily not only due to the wheel load of traveling vehicles but also due to the compound effects of freeze-thaw, chloride penetration, and alkali-silica reaction. Deteriorated concrete can break (Figure 1).
The applicability of the current technique for evaluating the durability of RC deck slabs is limited in that the evaluation technique is not suitable for every type of deterioration or damage, nor for every type of construction member. This limited applicability seems to be a factor in the wrong choices made for deterioration countermeasures and in the early re-deterioration of repaired or reinforced members. Because the deterioration of RC deck slabs can lead to reductions in traveling safety and to harm in people, a reasonable method for evaluating durability is needed toward the selection and implementation of appropriate countermeasures.
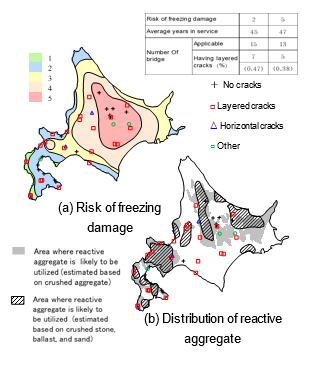
the risk of freezing damage and to the distribution of
reactive aggregate
Regarding the RC deck slabs of highway bridges in Hokkaido, the Structures Research Team of the Civil Engineering Research Institute for Cold Region has been collecting and analyzing data on bridges and deck slabs in order to clarify the characteristics of layered cracks within the slabs and of deteriorated concrete on the slab surface that has become friable (Figure 2). Although data are limited, the analysis results show the following: No definitive connection was found between the occurrence of deterioration and environmental factors, including the risk of freezing damage; deterioration and damage in the RC deck slabs of highway bridges have been reported from throughout Hokkaido (Figure 3); and the degree of deterioration or damage depends on the bridge alignment and waterproofing work. In the future, detailed analyses will address the mechanisms whereby the most common damages are generated, toward the proposal of a technique for durability evaluation.
(Contact: Structures Research Team, CERI)
Study on Disaster Prevention against Large Amount of Sea Ice floes and Debris Driven by Tsunami
Coastal waters in Hokkaido are partly covered with ice when sea ice floes reach the coast from northern part of Okhotsk Sea or when ice sheet grows in situ adjacent to the coast (land fast ice) in winter season. If a tsunami in ice-covered sea reaches the coast, run-up waves carrying ice may cause greater damage than regular waves due to their dynamic action occurring in ice-floe-laden flows; that is, pile-ups and jams of the ice floes may form in addition to the collision force by the ice floes.
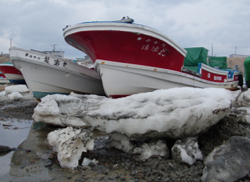
by the 2011 Off the Pacific Coast of Tohoku Earthquake
Several tsunami with ice events in the past involved damage caused by sea ice. One such event happened at the time of the Earthquake Off the Coast of Tokachi in 1952. On March 4, 1952, before the sea ice had gone, there was a strong earthquake off the coast of Tokachi with a Richter magnitude of 8.2. The tsunami due to the earthquake brought sea ice and caused serious damages. Sea ice and coastal ice cover were broken by the tsunami, and waves and large ice pieces ran up in urban areas, destroying houses and causing enormous damage. Regarding the 2011 Off the Pacific Coast of Tohoku Earthquake, damage to some facilities in coastal areas of Shikotan Island is considered to have been caused by a tsunami containing sea ice. At the same time eastern Hokkaido was attacked by tsunami waves as high as 3 m. Although most sea ice floes
fortunately had gone away the shore, some run-up
sea ice floes were found (Photo 1).
In December 2017, Japanese government´s committee on earthquake research announced a higher probability than previously estimated of a magnitude-9 megathrust earthquake that might occur off the Pacific coast of eastern Hokkaido in the following 30 years. In Hokkaido, ice cover develops and sea ice arrives at coastal areas mostly in the Sea of Okhotsk, but ice flows into the Pacific Ocean under certain conditions. In this regard, it is urgently necessary to take measures against tsunamis that might bring sea ice to coastal areas. We have been conducting research on countermeasures and have clarified the impacts of tsunami-borne sea ice on coastal areas and possible additional risks caused
by such ice.
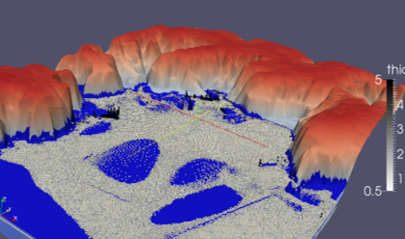
and sea ice run-up (The simulation technique is under
development; the height of sea ice pileup is shown
in gray scale.)
For example, in hydraulic model experiments, tsunamis are generated in water with floating models of sea ice. The behavior of the sea ice and the water level changes are investigated by examining sea ice pileups and ice jams (i.e., accumulation of, and blockage by, sea ice) (Photo 2). From lots of experimental results, we found the following simplified mechanisms. After the collision force imparted by the ice floes acts on structures, the tsunami flow is blocked by the formation of ice jams between the structures, and a large static force also acts on the structures because of the water level rise and hydrostatic pressure on the gaps between the structures (inter-structural spaces) due to the ice jam. We also developed a fundamental numerical simulation method of a run-up tsunami with ice floes by the use of a quasi-3D DEM (Discrete Element Method) in which phenomena unique to granular solids, such as the arch action, jam and pile up of such solids, can be simulated with a small computation load. This simulation technique will help to support the creation of hazard maps useful for evacuation planning. Various techniques will be utilized in the future for studying various factors in order to contribute to coastal disaster prevention.
(Contact: Port and Coast Research Team, CERI)