Study on a Technique for Using Boring Cores to Classify Serpentinite Properties
1. IntroductionIn constructing tunnels through mountains, geological surveys and geophysical explorations are conducted in advance to identify the rock mass characteristics of the mountains. Plans for excavation and falsework are designed based on these investigations. However, earth pressures greater than the resistance calculated for the falsework can develop during tunnel excavation, which sometimes leads to the collapse of the tunnel. Such great pressures tend to be most commonly generated when the mountain is distributed with serpentinite.
Serpentinite is a rock type that forms when peridotites from the deep mantle are altered to hydrous minerals (Fig. 1). The most common mineral constituents of serpentinite are the primary minerals that make up peridotite, such as olivine, pyroxene, and chrome spinel; secondarily serpentinized minerals such as serpentine group minerals, talc, and brucite, which are low-density hydrous minerals (i.e., water is incorporated in the crystal structure); and magnetite, which is ferromagnetic. Usually, the texture and composition ratios of these hydrous minerals significantly affect the physical properties of the rock, such as the hardness and the deformability. However, it is difficult to precisely understand and classify the constituent minerals of serpentinite through naked-eye observations or microscopy. Thus, various types of serpentinite have been addressed under the single name of ‘serpentinite’.
In this study, boring core samples from a tunnel excavation site in Hokkaido were used to develop a technique for classifying properties of serpentinite. Specifically, density, magnetic susceptibility, and quantitative ratios of hydrous minerals estimated by thermogravimetry were used for classification.
2. Research method
From boring core samples collected at a tunnel in Hokkaido, we cut out serpentinite specimens measuring about 1.5 cm x 1.5 cm x 1.5 cm. Density and magnetic susceptibility were measured by using a helium gas pycnometer and a magnetic susceptibility meter, respectively. Then, thermogravimetric changes (TG) in powderized specimens were measured by using a thermogravimetry/differential thermal analyzer (TG/DTA). The content of hydrous minerals (i.e., brucite and serpentine group minerals) was estimated based on the gravimetric changes from the dehydration of hydrous minerals. By using a DTG curve obtained through temperature differentiation of TG analysis results, peak-separation analysis was applied to overlapping dehydration reactions for the three types of serpentine group minerals (lizardite, chrysotile, and antigorite) to estimate the quantitative ratio of antigorite (i.e., high-temperature serpentine) in the serpentinite minerals (Fig. 2). The peak-separation analysis was performed by using an asymmetric Gaussian function (the exponentially modified Gaussian (EMG) function).
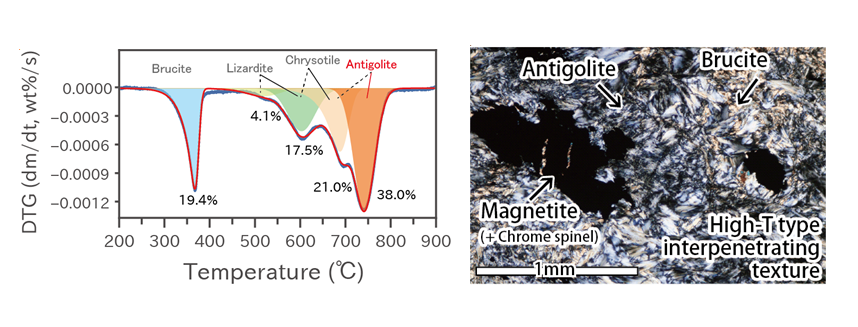
3. Results of the study
‘Serpentinite’, which has been treated as a single classification of rock, is known to have a distinctive distribution pattern in each producing area due to its density, which decreases with increase in serpentinization, and because of its magnetic susceptibility, which varies depending on the serpentinization conditions (lefthand chart in Fig. 3). The tendency in the changes of density and of magnetic susceptibility can be understood in relation to the strength and deformability of rock, and to its electrical conductivity. In addition to the relationship between the density and the magnetic susceptibility, a technique proposed by this study for quantifying the content of a high-temperature serpentine mineral (i.e., antigorite) in serpentinite, was used to classify the properties of serpentinite in greater detail than before (righthand chart in Fig. 3). It is necessary to examine whether the technique used in this study can be used to classify serpentinite into diverse mineral assemblages. However, it is hoped that the technique will help preliminary geological surveys and geophysical explorations to accurately estimate the strength of bedrock in tunnel excavation routes where serpentinite is distributed.
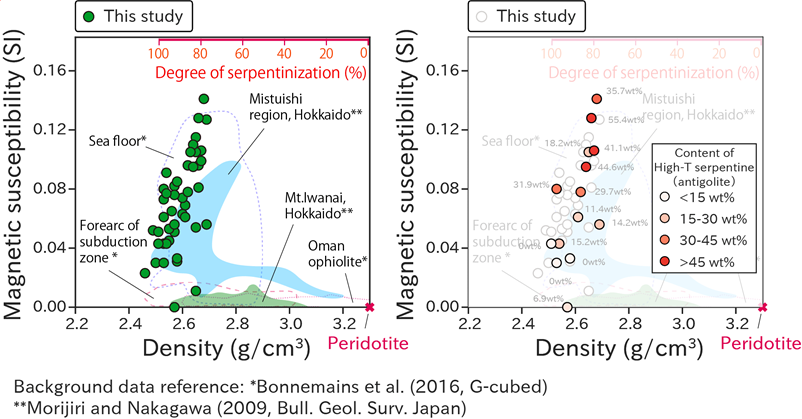
Right: Content of high-temperature serpentine (i.e., antigorite) estimated by using the proposed technique which helps classify the properties of serpentinite based on changes in magnetic susceptibility and density
(Contact : Geological Hazards Research Team, CERI)