
Improved Productivity of Concrete Block Retaining Walls Using Larger Blocks
Therefore, mainly for the purpose of saving labor, block products with the same depth but larger dimensions when viewed from the front have been developed and are used more widely. However, since each product is large, it is difficult to lay them in uncoursed masonry, which is the conventional laying method based on experience, and most products are laid in coursed masonry, which is a laying method of aligning the joints (Photo 1(b)).
The Construction Technology Research Team and the Japan Concrete Block Industries Association collected and statistically analyzed past earthquake damage cases in a joint study on the advancement of design, construction, and maintenance of large concrete block retaining walls (Aug. 2008 – Mar. 2021). The results confirmed that if wet masonry is performed correctly, there is no significant difference in damage between uncoursed masonry of wedge-shaped blocks and coursed masonry of larger blocks with a depth of 35 cm. A survey was also conducted of the actual products on the market and their structures, and block shapes and concrete placement methods were arranged to facilitate securing the quality of the construction of body-filling concrete, thereby proposing a method for building coursed masonry retaining walls that have equivalent performance to uncoursed masonry retaining walls, which are more difficult to construct than coursed ones. This is expected to promote the appropriate use of larger blocks, greatly improving workability and increasing productivity.
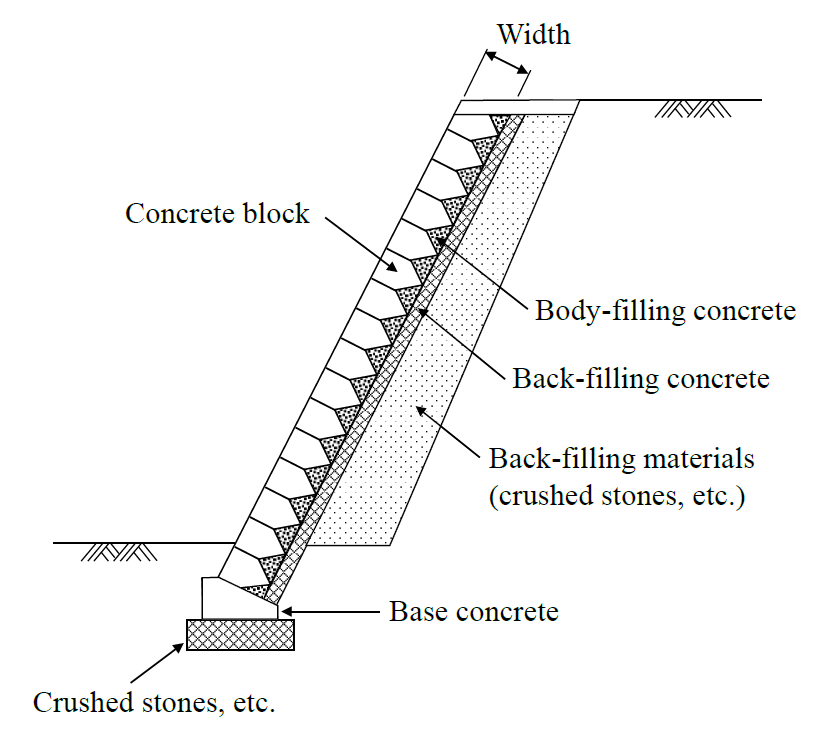
Figure 1 General structure of block
retaining walls
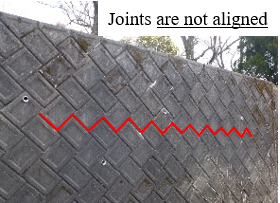
(a) Uncoursed masonry (in the case
of wedge-shaped blocks)
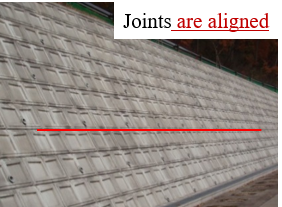
(b) Coursed masonry (in the case of
larger blocks with a width of 35 cm)
Photo 1 Concrete block retaining walls (examples)
(Contact: Construction Technology Research Team)
Management of Riverbeds to Prevent Bridges from Tilting
1. Introduction
In recent years, torrential rains that have been occurring in various areas have caused rivers to rise, resulting in damage such as tilted bridges, closed roads, and isolated settlements (Photo 1). It takes a long time to fix the tilted bridges and make the roads passable. Therefore, bridges must be managed so that road closures do not occur, even after flooding. PWRI is developing management methods that can prevent bridges from tilting due to rising river water (preventive maintenance).
2. Mechanism of bridge tilting due to rising river water
When river water flows torrentially, sand and stones on the river bottom (riverbed) may move and sometimes scrape the riverbed (riverbed degradation). If bridge piers are located in the river, the water will hit the front side of the piers, creating a downward flow. The downward flow may roll up sand and stones on the riverbed around the piers, causing deep scouring of the riverbed (local scour). As the riverbed degradation and local scour progress, the ground that supports the bridge (bearing layer) may be scraped away. As the bearing layer is scraped away, the ground’s ability to support the bridge (bearing capacity) decreases. Eventually, the ground can no longer support the bridge, causing it to tilt (Figure 1).
3. How should the riverbed be managed to prevent a bridge from tilting?
The new technology shown in Figure 2 makes it possible to efficiently measure the height of riverbeds over large areas. A comparison of riverbed heights measured at different times will reveal riverbed degradation and local scour. It is also possible to detect changes in the plane position of the deepest part of the river (changes in water flow paths), which is essential for predicting future riverbed degradation, as well as the appearance of sand and stone deposits on the surface of the river (sandbar formation). We are trying to prevent bridges from tilting by predicting, and responding to, future riverbed degradation and local scour using this type of river information that has not been adequately captured by bridge management in the past.
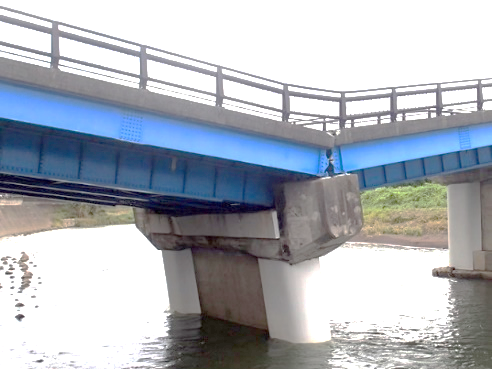
Photo 1 Example of a bridge that had tilted due to rising river water
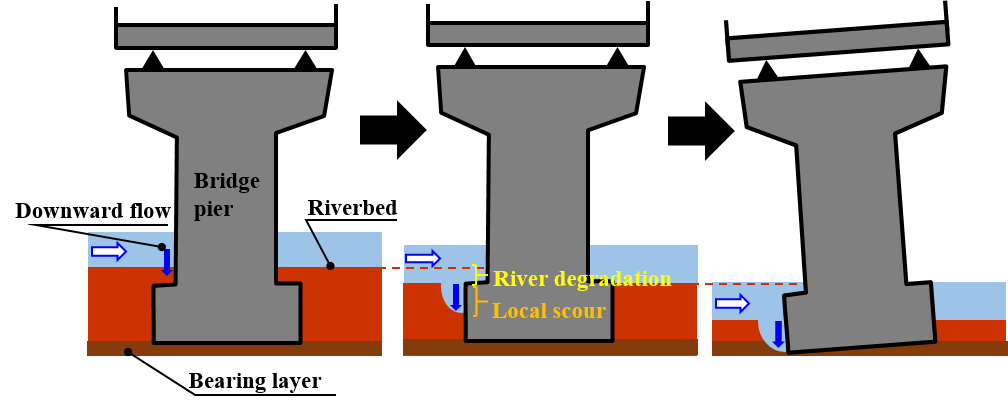
Figure. 1 Mechanism of bridge tilting (riverbed degradation and local scour)
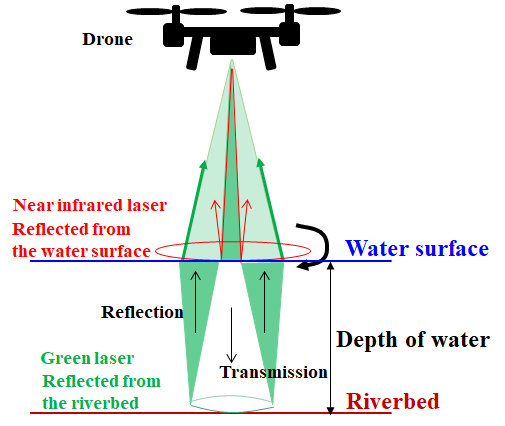
Figure 2: Example of new technology expected to be actively applied(Green laser & drone)
(Contact: Bridge and Structural Engineering Research Group)
Study on Resilience Technology of Road Bridges against Excess Action
During the seismic design of road bridges, structural details are determined not to exceed the critical state by examining the load-bearing capacity of each component through the use of the ocean trench and epicentral earthquake motions presented as earthquake motions that act on bridges in the Specifications for Highway Bridges. Since it is impossible to define the activity of unexpectedly large earthquakes, new ideas for handling large-scale disasters are necessary.
For the purpose of early restoration and securing the function of road bridges, the Structures Research Team is studying design methods that will allow avoidance of fatal damage caused by activity that exceeds the designed earthquake motion (Excess Action) and is looking into components that can realize such design. It specifically proposes a method of accurately elucidating the process of damage to bridges due to Excess Action through the use of load incremental analysis. Using this method, the team is conducting structural studies to determine the bearing capacity of bridge components that induce damage, based on the concept of bearing capacity differences of different components. Due to this damage induction, the bearing part is damaged before RC piers reach the limit state (state of ultimate failure) and avoid excessive force from being applied to the piers. The structure under consideration has a mechanism whereby the bolt part connects the lower shoe plate and base plate of the rubber bearing as shown in Figure. 2.This ensures the required length of the shear part and provides
the necessary firmness in combination with shear strength. An evaluation test of the damage induction function by cyclic loading using a pier model (Figure.3) allowed us to confirm that the induction mechanism properly. The team is planning to develop resilience technology in the future by studying other bridge types.
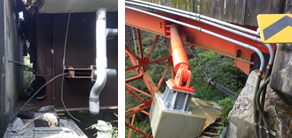
Figure. 1 A case of damage to some components with anti-catastrophe properties
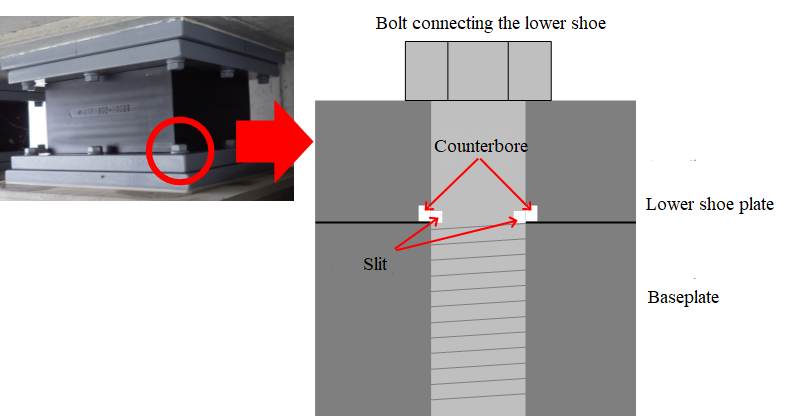
Figure. 2 Mechanism of the induction of damage of the bolt part connected to the lower shoe
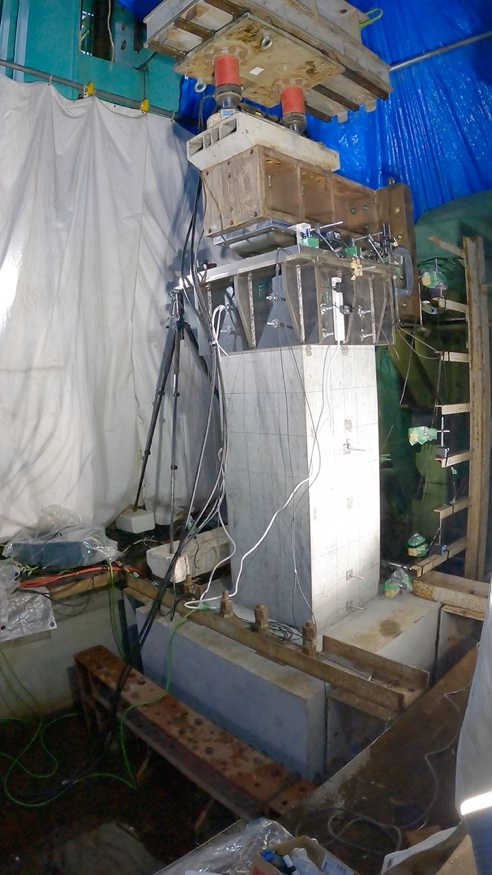
Figure. 3 Test to evaluate a component that
induces damage (with a load twice as large
(2δy) as the horizontal displacement at the time
of yielding of reinforcement at the outermost
edge of the pillar section)
(Contact: Structures Research Team, CERI)
Efficient Use of Recycled Asphalt Aggregate as a Pavement Base Course Material
1. Introduction
Asphalt pavement waste material has mainly been reused as aggregate for recycled hot asphalt mixture,
with the establishment of the Act on the Promotion of Effective Utilization of Resources in 1991 and the Construction Material Recycling Act in 2000.
There have also been surpluses in some regions when the used amount is smaller than the received amount.
Figure 1 shows the status of waste material at an intermediate treatment facility in the Wakkanai region.
The deposited amount of waste material was approximately 100,000 tons in FY 2020.
Although it is desirable to use the precious asphalt resources as aggregate in recycled hot asphalt mixture,
it is also important to utilize waste material without surplus in these regions. Therefore,
the team is conducting a study on technologies that can be applied to the use of waste material for the anti-frost layer of roadways and for its efficient use as a base course material of sidewalk pavement.
This article presents the study on its use as a base course material of sidewalk pavement.
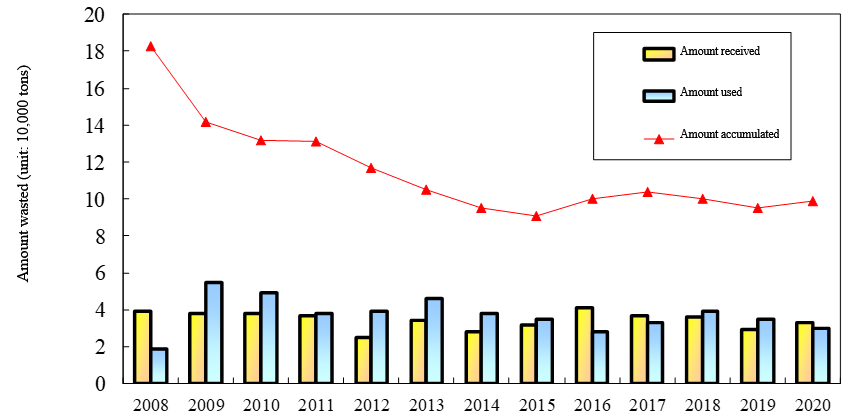
Figure.1 Use status of waste materials in the Wakkanai region
2. Evaluation through test construction
Test construction of a pavement base course material using recycled asphalt aggregate with a grain size that was adjusted to 0 to 40 mm and follow-up research were conducted in the Wakkanai region, and the following findings were obtained:
1) Compacting was performed successfully, and the required degree of compaction was achieved with recycled asphalt aggregate under the same construction conditions as for 40 mm class crusher-run stone, which is commonly used as a pavement base course material.
2) Figure 2 shows the results of bearing capacity evaluation using a DCP test at the time of construction. It was confirmed that recycled asphalt aggregate had the same or higher estimated CBR compared with that of 40 mm class crusher-run stone used for the construction section using it.
3) The results of bearing capacity evaluation after the road came into service using a small FWD and a simple bearing capacity gauge confirmed that the bearing capacity of recycled asphalt aggregate was equivalent to or better than that of 40 mm class crusher-run stone.
4) Figure 3 shows the results of measuring the road surface height in the traverse direction in the construction sections using recycled asphalt and crusher-run stone. There was less change in the road surface height in the section where recycled asphalt aggregate was used than in the section where crusher-run stone was used, indicating that frost heaving had not caused any unevenness. Visual inspection also confirmed that there were no cracks in the road surface.
The above-mentioned results suggested that there are no particular problems with the use of recycled asphalt aggregate as a pavement base course material and that it has the potential to be an effective means for promoting the use of pavement waste materials. The team is planning to continue follow-up surveys and study the long-term service properties and durability improvement effect of using pavement with recycled asphalt as the base course material.
Figure. 2 Evaluation of the bearing capacity
as a pavement base material
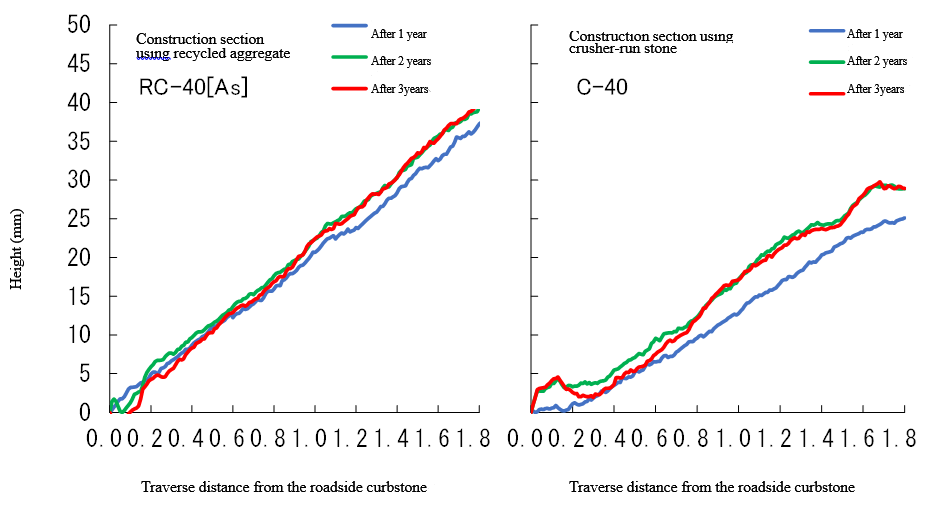
Figure. 3 Changes in road surface height after
construction using recycled aggregate
in this section
(Contact: Road Maintenance Research Team, CERI)